Basics of the Geostationary OrbitBy Dr. T.S. Kelso |
|
A geostationary orbit, also referred to as a geosynchronous equatorial orbit (GEO), is a circular geosynchronous orbit 35,786 kilometres (22,236 miles) above Earth's equator and following the direction of Earth's rotation. An object in such an orbit has an orbital period equal to the Earth's.
- The Long March 3B pitched away from Xichang, located in Sichuan province in southwestern China, on an easterly trajectory to head for an elliptical geostationary transfer orbit. Liftoff occurred.
- Geostationary Orbit refers to a circular orbit present above the earth’s equatorial plane possessing a period of revolution equivalent to the period of rotation of the earth. Here the term ‘geostationary’ corresponds to the orbit where the satellite appears to remain fixed at a point or stationary with respect to the surface of the earth.
- 2 days ago Geostationary satellites orbit 22,236 miles above the equator at speeds equal to Earth’s rotation. This means they continuously view the same area. Because they stay above a fixed spot on the surface, they provide constant vigil to identify and track severe weather conditions.
- The geostationary orbit is a special case of the geosynchronous orbit, which is any orbit with a period equal to Earth’s rotation period. The concept for such an orbit was proposed in 1945 by British author and scientist Arthur C. Clarke in an article entitled “Extra-Terrestrial Relays” for Wireless World.
| |
Basics of the Geostationary OrbitBy Dr. T.S. Kelso |
Few aspects of the Space Age have had as much impact on our everyday lives as the invention of the communications satellite. In just a few short decades, they have brought together even the most far-flung reaches of the globe in ways that not that long ago were barely imaginable. In fact, today it is possible to talk directly to climbers at the top of Mount Everest or communicate via the Internet with virtually any computer system on the face of the planet—all with the help of communications satellites.
While communications satellites perform their missions in many types of orbits, from near-earth constellations like Iridium and Globalstar to the highly-inclined, eccentric Molniya orbits used by the Russian Federation, one of the more important classes of orbits for these satellites is the geostationary orbit. In this column, I'd like to examine the unique aspects of this class of orbit which make it suitable for not only satellite communications, but early warning and weather observations, too.
History
The concept of the geostationary orbit has been around since the early part of the twentieth century. Apparently, the concept was originated by Russian theorist Konstantin Tsiolkovsky—who wrote numerous science and science-fiction articles on space travel at the turn of the century. In the 1920s, Hermann Oberth and Herman Potocnik—perhaps better known by his pseudonym, Herman Noordung—wrote about space stations which maintained a unique vantage over the earth.1 Each author described an orbit at an altitude of 35,900 kilometers whose period exactly matched the earth's rotational period, making it appear to hover over a fixed point on the earth's equator.
However, the person most widely given credit for the concept of using this orbit for communications is Arthur C. Clarke. In an article he published in Wireless World in October 1945 titled 'Extra-Terrestrial Relays: Can Rocket Stations Give World-wide Radio Coverage?' Clarke extrapolates from the German rocket research of the time to a day when communications around the world would be possible via a network of three geostationary satellites spaced at equal intervals around the earth's equator (see Figure 1).
Figure 1. Original figure from Clarke's article in the October 1945 edition of Wireless World2
In this article, Clarke not only determines the orbital characteristics necessary for such an orbit, but also discusses the frequencies and power needed for communications to and from space and how to use solar illumination for power—he even calculates the impact of solar eclipses around the vernal and autumnal equinoxes. What makes this article all the more remarkable is that Clarke wrote it more than a dozen years before the first satellite was even launched.
It wasn't until 1963 that NASA set out to test Clarke's concept with the Synchronous Communications Satellite program. Unfortunately, Syncom 1—launched 1963 February 14—while successfully reaching geosynchronous orbit in an inclined, eccentric orbit was unsuccessful due to an electronics failure. Syncom 2—launched 1963 July 26—became the first operational geosynchronous communications satellite. Syncom 3—launched 1964 August 19—became the first geostationary satellite, finally fulfilling the prediction made by Clarke almost twenty years earlier.
Theory
So just what is a geostationary orbit? In general terms, it is a special orbit for which any satellite in that orbit will appear to hover stationary over a point on the earth's surface. Unlike all other classes of orbits, however, where there can be a family of orbits, there is only one geostationary orbit. Let's examine this orbit's unique characteristics.
For any orbit to be geostationary, it must first be geosynchronous. A geosynchronous orbit is any orbit which has a period equal to the earth's rotational period. As we shall soon see, this requirement is not sufficient to ensure a fixed position relative to the earth. While all geostationary orbits must be geosynchronous, not all geosynchronous orbits are geostationary. Unfortunately, these terms are often used interchangeably.
Before continuing, it is necessary to clarify what is meant by 'the earth's rotational period.' For most timekeeping, we consider the earth's rotation to be measured relative to the sun's (mean) position. However, since the sun moves relative to the stars (inertial space) as a result of the earth's orbit, one mean solar day is not the rotational period that we're interested in. A geosynchronous satellite completes one orbit around the earth in the same time that it takes the earth to make one rotation in inertial (or fixed) space. This time period is known as one sidereal day and is equivalent to 23h56m04s of mean solar time (for more information, see 'Orbital Coordinate Systems, Part I' in the September/October 1995 issue of Satellite Times). Without any other influences, the earth will be oriented the same way in inertial space each time a satellite with this period returns to a particular point in its orbit.
To ensure that a satellite remains over a particular point on the earth's surface, the orbit must also be circular and have zero inclination. Figure 2 shows the difference between a geostationary orbit (GSO) and a geosynchronous orbit (GEO) with an inclination of 20 degrees. Both are circular orbits. While each satellite will complete its orbit in the same time it takes the earth to rotate once, it should be obvious that the geosynchronous satellite will move north and south of the equator during its orbit while the geostationary satellite will not.
Figure 2. Geostationary and Geosynchronous Orbits
Orbits with non-zero eccentricity (i.e., elliptical rather than circular orbits) will result in drifts east and west as the satellite goes faster or slower at various points in its orbit. Combinations of non-zero inclination and eccentricity will all result in movement relative to a fixed ground point.
Figure 3 shows some typical results. The figure-eight ground track is that of the geosynchronous orbit (GEO) shown in Figure 2. The geostationary satellite (GSO) sits fixed at the crossover point of the figure eight (over the equator). If we now give the geosynchronous satellite an eccentricity of 0.10, the slanted teardrop shape results. Typically, eccentric geosynchronous orbits will result in a slanted figure eight—this one just happens to have the crossover point at the northern apex of the ground track.
Figure 3. Geosynchronous Ground Tracks
It should now be apparent that only satellites which orbit with a period equal to the earth's rotational period and with zero eccentricity and inclination can be geostationary satellites. As such, there is only one geostationary orbit—a belt circling the earth's equator at an altitude of roughly 35,786 kilometers.
It should also be clear that it is not possible to orbit a satellite which is stationary over a point which is not on the equator. This limitation is not serious, however, since most of the earth's surface is visible from geostationary orbit. In fact, a single geostationary satellite can see 42 percent of the earth's surface and a constellation of geostationary satellites—like the one Clarke suggested—can see all of the earth's surface between 81° S and 81° N.
Of course, the advantage of a satellite in a geostationary orbit is that it remains stationary relative to the earth's surface. This makes it an ideal orbit for communications since it will not be necessary to track the satellite to determine where to point an antenna. However, there are some disadvantages. Perhaps the first is the long distance between the satellite and the ground. With sufficient power or a large enough antenna, though, this limitation can be overcome.
The fact that there is only one geostationary orbit presents a more serious limitation. Just as in putting beads on a loop of string, there are only so many slots into which geostationary satellites can be placed. The primary limitation here is spacing satellites along the geostationary belt so that the limited frequencies allocated to this purpose don't result in interference between satellites on uplink or downlink. Of course, we also want to make sure the satellites aren't close enough to run into one another since they will have some small movement.
While new communications satellites may be placed in a true geostationary orbit initially, there are several forces which act to alter their orbits over time. Since the geostationary orbital plane is not coincident with the plane of the earth's orbit (the ecliptic) or that of the moon's orbit, the gravitational attraction of the sun and the moon act to pull the geostationary satellites out of their equatorial orbit, gradually increasing each satellite's orbital inclination. In addition, the noncircular shape of the earth's equator causes these satellites to be slowly drawn to one of two stable equilibrium points along the equator, resulting in an east-west libration (drifting back and forth) about these points.
To counteract these perturbations, sufficient fuel is loaded into all geostationary satellites to periodically correct any changes over the planned lifetime of the satellite. These periodic corrections are known as stationkeeping. North-south stationkeeping corrects the slowly increasing inclination back to zero and east-west stationkeeping keeps the satellite at its assigned position within the geostationary belt. These maneuvers are planned to maintain the geostationary satellite within a small distance of its ideal location (both north-south and east-west). This tolerance is normally designed to ensure the satellite remains within the ground antenna beamwidth without tracking.
Once the satellite has exhausted its fuel, its inclination will begin to grow and it will begin to drift in longitude and may present a threat to other geostationary satellites. Oftentimes, geostationary satellites are boosted into a slightly higher orbit at the end of their planned lifetime to prevent them causing havoc with other geostationary satellites. This final maneuver assumes that no unplanned failure has occurred which would prevent it (such as a power or communications failure).
Summary
This initial article on geostationary and geosynchronous orbits should give you a basic understanding of some of the fundamental orbital concepts. In our next column, I would like to continue this topic by examining the relationship among the observer, satellite, and the sun to determine a geostationary satellite's longitude, the look angles from a terrestrial observer, and how the position of the sun can affect onboard power management and interference with satellite communications.
As always, if you have any questions, please feel free to write me at TS.Kelso@celestrak.com. Until next time, keep looking up!
Notes
1 Oberth, Hermann. Die Rakete zu den Planetenraumen (The Rocket into Interplanetary Space), 1923. Noordung, Herman. Das Problem der Befahrung des Weltraums (The Problem of Space Travel), 1929.
2 Clarke, Arthur C. 'Extra-Terrestrial Relays: Can Rocket Stations Give World-wide Radio Coverage?' Wireless World, October 1945, p. 306.
What is the difference between geosynchronous and geostationary orbits?
There’s a sweet spot above the Earth where a satellite can match the same rotation of the Earth.
Geostationary Orbit Altitude
This special position in high Earth orbit is known as a geosynchronous orbit.
But how is this any different from a geostationary orbit?
Let’s dive into some of the differences between geosynchronous and geostationary orbits.
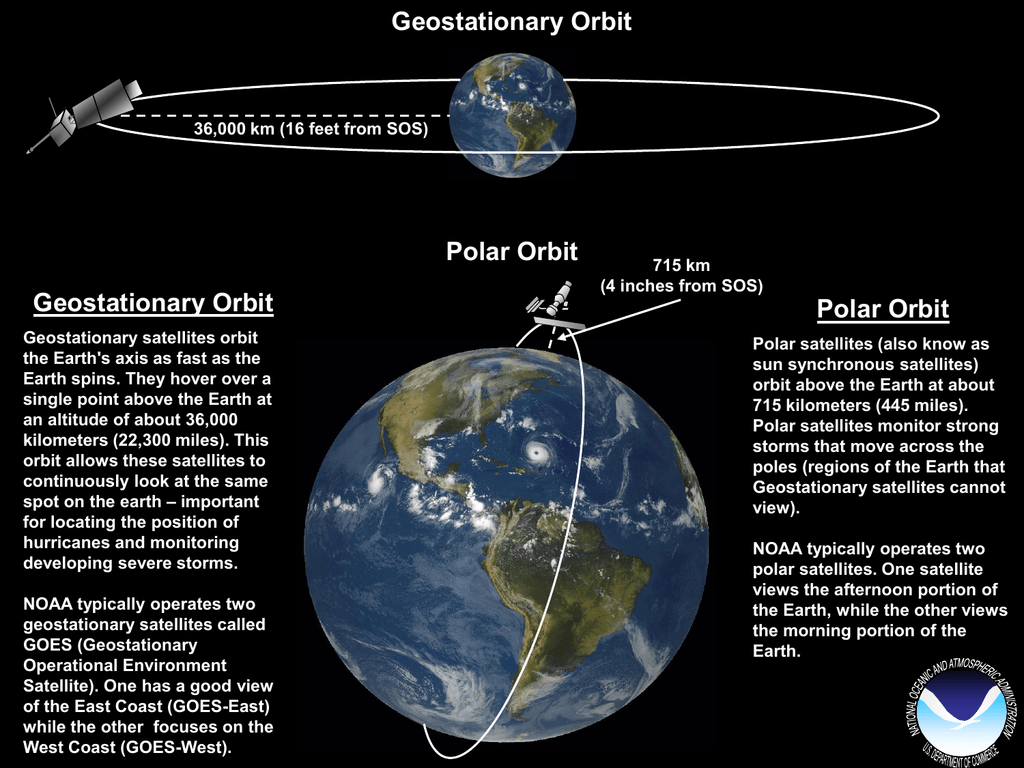
Geosynchronous Orbit
About 35,786 kilometers above the Earth’s surface, satellites are in geostationary orbit. From the center of the Earth, this is approximately 42,164 kilometers. This distance puts it in the high Earth orbit category.
At any inclination, a geosynchronous orbit synchronizes with the rotation of the Earth. More specifically, the time it takes for the Earth to rotate on its axis is 23 hours, 56 minutes, and 4.09 seconds, which is the same as a satellite in a geosynchronous orbit.
If you are an observer on the ground, you would see the satellite as if it’s in a fixed position without movement.
This makes geosynchronous satellites particularly useful for telecommunications and other remote sensing applications.
Geostationary Orbit
While geosynchronous satellites can have any inclination, the key difference to geostationary orbit is the fact that they lie on the same plane as the equator.
Geostationary orbits fall in the same category as geosynchronous orbits, but it’s parked over the equator. This one special quality makes it unique from geosynchronous orbits.
Weather monitoring satellites like GOES are in geostationary orbits because they have a constant view of the same area. In a high Earth orbit, it’s also useful for search and rescue beacons.
Here’s how both orbits compare:
While the geostationary orbit lies on the same plane as the equator, the geosynchronous satellites have a different inclination.
This is the key difference between the two types of orbits.
Geostationary Orbit Speed
Semi-Synchronous Orbit
Global Positioning System (GPS) satellites are in another sweet spot known as semi-synchronous orbits. While geosynchronous orbits match the rotation of Earth (24 hours), semi-synchronous orbits take 12 hours to complete an orbit.
Instead of 35,786 kilometers above the Earth’s surface, semi-synchronous orbits are approximately 20,200 kilometers above the surface. This puts them in the medium Earth orbit range out of the three classes of orbits.
These orbits are close to zero in eccentricity, meaning they are near-circular. Eccentric orbits define how stretched orbits are. The closer eccentricity is to zero, the more the orbit closer to a circle. The closer to one, the orbit becomes longer and skinnier.
Do you want to learn more about satellites?
For the 21st century and beyond, satellites will play an important role in some of the fundamental challenges.
Not to mention, the physics of satellite orbits are remarkable. And they have many practical purposes for science and innovation.
Geostationary Orbit Gso
If you want to build on your expertise in satellite orbits, here a few more resources to expand your knowledge
